Central Nervous System of Insects
昆虫の体制と神経系
昆虫の体は連続する十数個の体節から構成され,頭部・胸部・腹部の三つの部位に区別される.そのうち頭部は前方の6個の体節が融合したもので,第1体節には複眼,第2体節には触角が発達している.頭部に続く三つの体節が胸部(前胸・中胸・後胸)で各体節に1対の肢が付属し,多くの昆虫では中胸と後胸からは前翅と後翅がそれぞれ1対づつ派生している.腹部は11ないし12の体節からなるが,融合により8~9節となっている場合が多い.
昆虫の神経系は,神経節の連鎖からできている中枢神経系と,消化管などの内臓筋を支配する口胃神経系を含む末梢神経系から構成される.さらに昆虫の神経系には,脳間部-側心体-アラタ体系や,貯蔵放出器官などから構成される神経内分泌系が含まれている.
中枢神経系は,基本構造としては梯子状をおり,ハシゴ状神経系といわれる.はしごの2本の長い材(縦方向)に相当する1対の縦連合が体軸と平行に走行し,横木に対応する部分に神経節が存在する.(発生学的には,外胚葉由来の神経節原基が各体節に1対ずつ形成され,左右の神経節原基が融合して神経分節となり,神経分節から前後に軸索の束が伸びて縦連合が形成される.)
最も前方に位置する脳神経節(単に脳ともいう)は食道より前方の3個の神経分節が1つに融合したもので,食道上神経節ともいう.[[食道下神経節]]は,食道より後方の3個の神経分節が融合したものである.脳神経節と[[食道下神経節]]の間の縦連合の間を食道が貫通する.さらに後方には,[[胸部神経節]],[[腹部神経節]]と続く.[[食道下神経節]]から後方の神経節は消化管の下側(腹側)に並んでいて,腹側神経索あるいは腹側神経節連鎖とよばれる.脊椎動物の脊髄は消化管の上側(背側)にあり,昆虫の神経索の配置とは逆になっている.これはない骨格と外骨格の違いによるもので,脊椎動物では脊髄が入った脊椎骨で内臓をつる体制になっているのに対し,昆虫では神経索や内臓をクチクラでできた腹板で下から支えた体制となっている.
神経節の融合は,昆虫の分類群に特異的な神経系の系統発生を反映しており,実にさまざまな融合パターンがある.たとえばミツバチ,ハエ,ガ等では、脳神経節と[[食道下神経節]]が融合していて,これを食道が貫通している.また,バッタの胚では腹部体節に11対の神経節原基が出現するが,発生過程で第1から第3番目の[[腹部神経節]]は後胸神経節と融合し,さらに第8から第11番目の[[腹部神経節]]が融合して大型の最終[[腹部神経節]]を形成する.ハエでは胸部と腹部の神経節が融合して分節構造をもった一つの塊となっている.
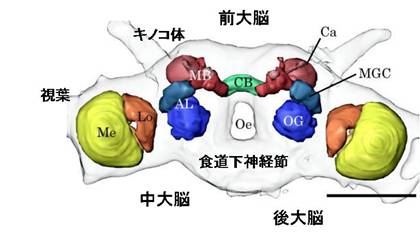
図:カイコガの脳。脳は前大脳、中大脳、後大脳から構成される。脳内には、神経が集まり神経情報を処理する集合体(神経叢)ある。代表的な神経叢は、前大脳の[[視葉]]、キノコ体、前大脳側部、中心体、そして中大脳の[[触角葉]]である。脳の下部には[[食道下神経節]]がつながる。スケールは0.5mm。
神経節の基本構造
神経節の表面は[[神経鞘]]覆われ,内部の神経組織は機械的および化学的に保護されている.[[神経鞘]]の外側は細胞外物質からなる神経鞘皮で,内層は周膜細胞(グリア細胞の一種)が単層に並んだ神経周膜である.神経鞘皮が機械的に,神経周膜が血液-脳関門として化学的に神経組織を保護していると考えられている.
神経節の神経組織は,神経細胞([[ニューロン]])の細胞体が神経節表層に集まった皮層と,その内側の[[神経叢(ニューロパイル)]]とに明確に二分されている.昆虫の神経細胞は普通単極性であり,細胞体からは,まず1本の神経突起が神経叢に伸びて,その先でさまざまな形態の分子を広げ,シナプスを形成する.したがって皮層では細胞体にシナプスが形成されることはなく,シナプスはすべて神経叢にあると考えられている.
[[神経叢(ニューロパイル)]]は密集神経叢,非密集神経叢,神経路に区別される.密集神経叢は細かく分枝した神経突起が密にからまって高密度でシナプスを形成している領域で,周りとの境界が比較的明瞭であり,グリア細胞の層で境界されていることも多い.非密集神経叢はシナプスの密度が低く,境界も不明瞭な領域で,一般染色では明瞭な内部構造は見られない.神経路は神経叢をつなぐ軸索の束である.相当数の軸索が密集して束を形成する場合には,周囲との境界は明瞭だが,小数の軸索からなる束や,束がほつれている場合などには,周囲との厳密な区別はつけにくい.
密集神経叢は,球状,円柱状,または層板状などの,さらに小さい多数のモジュール構造にわかれていることが多い.モジュールといえば,脊椎動物大脳皮質のカラムが想起されるが,カラムは機能的に区別できるものの,形態学的には区画されて見えるわけではない.一方,昆虫の場合は機能モジュールであると同時に,形態学的にも明瞭な区画化がなされており,研究上の大きな利点となっている.
モジュールは,互いによく似た微小回路であり,それらが多数集合して並列分散処理を行う.たとえば,[[視葉]]のモジュールは複眼の1個の個眼からの情報を処理し,[[触角葉]]のモジュールはある1種類の嗅受容タンパク質を発現している受容細胞群からの情報を処理する.つまり,ある密集神経叢が特定の感覚種機能分担しているのに対して,その中の個々のモジュールは,その感覚種の中のある特定の質にかんする情報処理を分担している.
脳の構造と機能分担
昆虫の脳は,105から106個の神経細胞から構成され,その数は腹側神経索を構成するすべての神経節に含まれる神経細胞の総数よりも多い.イエバエの脳には約35万個,ミツバチ(働きバチ)の脳には約85万個の神経細胞が存在している.一方,[[胸部神経節]]は3000~5000個の神経細胞から構成され,またハシゴ状に連なる[[腹部神経節]]のそれぞれは,昆虫種によりことなるが,400~850個の神経細胞から構成されている.
脳は前大脳 (protocerebrum),中大脳 (deutocerebrum),後大脳 (tritocerebrum)の三つの領域に分けられる.それぞれは,頭部第1~第3節の神経分節に由来する.前大脳の両側には大きく張り出した[[視葉]]がある.[[視葉]]は3または4個の神経叢から構成されている.左右の[[視葉]]にはさまれた前大脳中央域には,[[キノコ体]]と呼ばれる1対の神経叢がある.さらにキノコ体にはさまれた前大脳正中部には不対の神経叢である前大脳橋や中心体があり,それらをあわせて[[中心複合体]]と呼ぶ.これらの密集神経叢の形や大きさ,配置は昆虫種により若干ことなるが,共通点も多い.[[視葉]]は視覚情報の処理,キノコ体は匂いの学習や記憶の形成など,中心複合体は行動出力の企画・選択・修飾などに,それぞれ関係していると考えられている.これらの密集神経叢の間や周囲には,前大脳側葉,[[側副葉]],後傾斜などの非密集ニューロパイルが存在する.
中大脳で顕著な神経叢は1対の[[触角葉]]で,ここには主として触角に分布する嗅受容細胞の軸索が触角神経を経て投射している.[[触角葉]]の後方には,触角にある機械受容細胞の軸索が投射する背側葉がある.触角を動かす筋肉を支配する運動神経細胞も背側葉に分布していることから,この神経叢は機械感覚-運動中枢とも呼ばれる.
後大脳は脳でもっとも小さな領域で特徴的な構造をもつ神経叢は見られない.後大脳は縦連合により後方の[[食道下神経節]]とつながり,また前額神経節は,心臓や消化管の内臓筋を支配する口胃神経系の中枢であり,回帰神経を経て[[食道下神経節]]とつながる.
[[食道下神経節]]は,主に口器の感覚と運動を司る.すなわち,口器は小顎鬚(しょうがくしゅ)や唇弁など,さまざまな付属肢によって構成されているが,そこに 分布する感覚子の受容細胞の軸索が[[食道下神経節]]に終末する.また,触角上の感覚子のうち味覚の感覚子の受容細胞は中大脳だけでなく[[食道下神経節]]にも終末する.
[[胸部神経節]]には飛翔,歩行,発音などの重要な行動を制御する運動中枢がある.[[腹部神経節]]には,姿勢保持や,呼吸・循環に不可欠な腹部のリズミカルな運動,さらに交尾行動や産卵行動の制御に関わる運動中枢がある.これらの運動中枢は,それぞれに特有な感覚入力(トリガー入力)によって,脳と独立して自律的に運動パターンを発現したり補正しているが,行動の開始や維持などは脳からの下降性出力によって制御されている.
前大脳の高次神経叢であるキノコ体,中心複合体,前大脳側葉には,[[視葉]]や中大脳。後大脳,[[食道下神経節]]や腹側神経索からの感覚情報が,何層かのフィルターを通して処理・統合された形で伝えられる.高次神経叢では,種々の感覚種の情報が処理。統合され,最終的な決定は下降性介在神経の出力に変換される.この下降性の出力によって腹側神経索にある運動中枢が調節去れ,個体が直面しているさまざまな状況に対して適切な反応や行動を生み出す.したがって,頭部と腹部を切り離し,胸部だけの状態でも,羽ばたきや歩行を起こすことができる.また,[[胸部神経節]]にも学習能力がある.断頭したゴキブリを吊り下げ,脚がある高さまで下がると電気ショックが与えられるようにすると,30から40分でこのゴキブリは脚を次第に下げなくなる.このように昆虫では学習が[[胸部神経節]]など脳以外でも起こる.
腹部神経節
昆虫の脳を構成するニューロン
昆虫の脳は,[[ニューロン]](neuron)とグリア(glia)といわれる細胞からなる.[[ニューロン]]もグリア細胞ももとは同じ細胞(幹細胞)から分化する.グリア細胞は[[ニューロン]]の構造の維持など[[ニューロン]]のサポート役として機能する.そして,脳の働き(情報処理)の中心は[[ニューロン]]である.「神経細胞」ともいう.[[ニューロン]]というのはギリシア語の「すじ」というのが語源である.
哺乳類では繰りのほうが神経細胞の10倍以上多いとされるが,昆虫では逆にグリア細胞は神経細胞の10%程度である(キイロショウジョウバエの結果:文献1)
[[ニューロン]]の基本的な構造と機能は動物を通して共通している.[[ニューロン]]が回路をつくって情報処理をするような神経系は,イソギンチャクやクラゲなどの刺胞動物ではじめて現れ,次第に複雑な神経回路からなる脳を獲得していった.昆虫は下等だから,高等なヒトとはちがった[[ニューロン]]からできているということはない.ただ,昆虫の寸法は,センチメートル程度である.したがって,脳のサイズも小さくなり,昆虫の脳をつくる[[ニューロン]]の数は哺乳動物の脳に比べて桁違いに少なくなる.ヒトの脳が1000億なのに対して,昆虫の脳はわずかに10万から100万個である.
昆虫の神経細胞は,大きく分けて次の5種類に分類される.
1)感覚神経:末梢から中枢神経系に投射投射する神経細胞
2)[[投射神経(出力神経)]] /FONT>:中枢神経系内の離れた2つの領域を接続する神経細胞
3)局所神経:中枢神経系の1つの領域内のみで終わる神経細胞
4)運動神経:中枢神経系から筋肉に投射する神経細胞
5)神経分泌細胞:内分泌器官などに投射する神経細胞
1)-5)のうち,1)感覚神経のみその細胞体が末梢にあり,他は脳の表層(皮質 rind cortex)に集中している.これは昆虫脳のおおきな特徴である.これは哺乳類脳の大脳皮質に似ているが,大脳皮質では皮質内部に多数のシナプスが存在するのに対し,昆虫脳では皮質にシナプスは一切存在しない.神経細胞は,皮質から脳の内部神経線維を伸ばし,ニューロパイルでシナプスを形成する.神経叢内にはシナプスはない(図9).
哺乳類では多くの神経線維はグリアにより覆われた有髄神経であるが,昆虫では無髄神経が多く,グリアは多数の神経線維の束をまとめて覆っていたり,神経叢の区画を多い,境界を形成することが多い.
昆虫の脳をつくる個々の[[ニューロン]]はその形とはたらきによって特徴付けられるものが多く,「同定ニューロン(identified neuron)」といわれる(図3).昆虫の脳の研究は,このような「少数」,「同定」という特徴を活かして単一のニューロンレベルからその素性を明らかにしながら,脳を理解するという方法がとられてきた.
神経細胞で情報を受ける樹状突起は,哺乳類では細胞体から直接伸びているが,昆虫では神経線維から側枝として伸びる.シナプスは形態的に神経線維形態から推定ができるものが多い.棘状(spine)は入力v,コブ状(bouton,varicose)に膨らんだ場合には出力シナプスである場合が多い.また,細胞体に近い部分には入力シナプス,遠い部分には出力シナプスが多い傾向があるが,一部逆の場合もある.
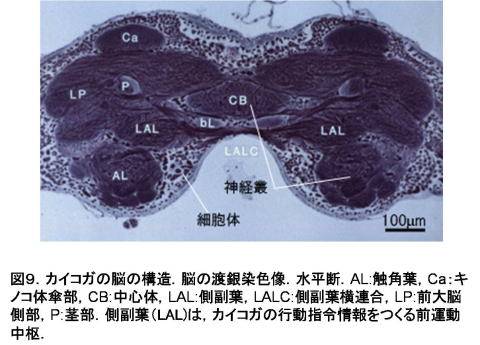
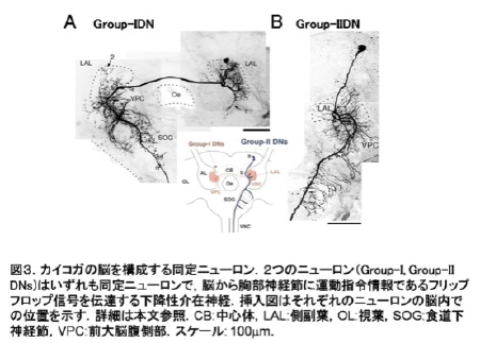
昆虫脳をつくるニューロンのかたち
図8に昆虫の脳をつくる[[ニューロン]]の例を示した.核をもつ球状の構造を細胞体という.大きさは10ミクロン(0.01ミリメートル)くらい.細胞体からは突起がでているが,それが一本のものを単極細胞(例:図8),二本のものを双極細胞,たくさん突起をもつものを多極細胞という.昆虫の[[ニューロン]]では,細胞体から一本の線維が出て,それが複雑に分かれる単極のものがほとんど.突起の中でもひときわ長いものがある.これを軸索という.軸索は[[ニューロン]]の情報を次の[[ニューロン]]に伝えるための伝導路.そして軸索の末端部は,次に情報を伝える[[ニューロン]]に接続される.この接続部をシナプスという.
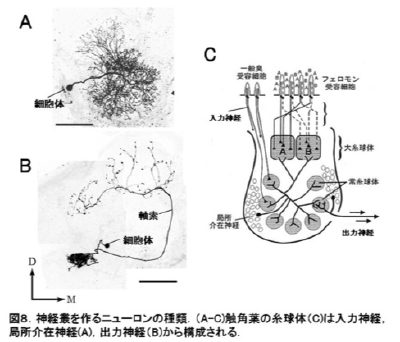
参考文献
1.昆虫ミメティクス (p126)
Central Nervous System of Insects
Body plan and nervous system of insects
The insect body consists of a number of consecutive segments and is differentiated into three parts: the cephalon (head), the thorax, and the abdomen . The cephalic part is fused from the anteriormost six segments. The compound eyes belong to the first segment, the antennae to the second segment. The third segment bears the labrum as an appendage. The other three segments are associated with the mandibles, the maxillae, and the labium. The next three segments form the thoracic part. A pair of limbs is attached to each of the segments. The first thoracic segment is the prothorax. In many insect species, a pair of anterior and posterior wings is attached to the second thoracic segment (mesothorax) and third segment (metathorax) respectively. The abdominal part consists of eleven or twelve segments but in many cases they are fused into eight or nine segments.
The insect nervous system comprises the central nervous system and the peripheral nervous system. The central nervous system consists of a chain of ganglia. The peripheral nervous system includes a stomatogastric ganglion and sensory and motor nerves. In addition, insect nervous system includes pars intercerebralis-corpus cardiacum-corpus allatum and neuroendcrine system that consists of store and release organ.
The structure of the insect central nervous system resembles that of a rope ladder, therefore, it is also called ladder-like nervous system. The stiles of a ladder correspond to the connectives, running aligned to the body axis (except in the head, where the neuraxis is often bent). A ladder rung corresponds to a ganglion with commissures. (The CNS can be considered to be an ectodermal rudiment)
The most anterior ganglion is the cerebral ganglion (also supraoesophageal ganglion or brain), a structure that evolved from the fusion of the three preoral neuromeres (anterior with respect to the oesophagus). The suboesophageal ganglion consists of the fusion of three postoral neuromeres. The oesophagus is surrounded by the circumoesophageal connectives that link supra- and suboesophaegeal ganglia. More posterior to them are thoracic ganglia and abdominal ganglia. Ganglia posterior to the supraoesophagial ganglion lie ventral with respect to the digestive tract and are, with the exlusion of the suboesophageal ganglion, also called ventral nerve cord. In comparison, the vertebrate spinal cord lies dorsal with respect to the digestive tract. This difference is derived from their skeleton, each of which is external skeleton and internal skeleton. Vertebrate has a body plan that vertebral column puts up visceral organ whereas invertebrate has a body plan that ventral plate puts up nerve cord and visceral organ.
The variety of fusion patterns of ganglia reflects the phylogeny of taxonomic groups at the level of the CNS. For example, honey bee, fly, and moth have a fused form of brain and suboesophageal ganglion with an oesophageal foramen, through which the oesophagus extends towards the mountparts. The locust embryo has eleven pairs of ganglion rudiments. During ontogeny, the first to third abdominal ganglia fuse with the metathoracic ganglion and the eighth to eleventh ganglia fuse to and form a large terminal abdominal ganglion. In Diptera, fusion of thoracic and abdominal ganglia occurs resulting in a large segmented agglomerate ganglion
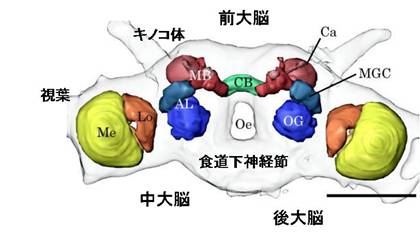
Figure: Silkmoth brain (male). The silkmoth brain consists of protocerebrum, deutocerebrum, and tritocerebrum. The brain has many identifiable regions consisting of densely packed neurites of the constituent neurons: the neuropils. Information processing occurs mostly in the neuropil. . Representative neuropils are the optic lobes (in principle, this would have to be divided), mushroom bodies, the lateral protocerebrum, and the central body in the protocerebrum, and the antennal lobe in the deutocerebrum. Ventrally, the brain is fused with the suboesophgeal ganglion. Scale bar: 0.5 mm.
Basic structure of ganglia
Ganglia are externally delimited by an insulating cell layer, the neurolemma, forming a chemical and mechanical barrier. Outer layer of the neurolemma is called neural lamella (or sheath) that is composed of extracellular matrix produced by an inner layer, the perineurial sheath cell layer consisting of glia cells. The lamella protects the neuronal tissue mostly mechanically while the sheath cell layer acts as a blood-brain barrier, being relatively impermeant as demonstrated by lanthanum ion exposure.
Neuronal tissue within a ganglion is grouped into two layers, an outer soma layer and an inner neuropil core. Insect central neurons are usually monopolar cells. A single neurite extends from the soma and branches into several neurites, forming synapses with adjacent neurites of other neurons. Generally, the majority of synapses are localised in the neuropil.
The neuropil is classified into glomerular neuropil, non-glomerular neuropil, and neuronal tracts. Glomerular neuropil is a region where finely branched neurites form dense tangles and numerous synapses. The border of dense neuropil is clearly discernable and often delineated by a glia cell layer. non-glomerular neuropil has a low synapse density and poorly defined borders. Its internal structure can not be revealed with general staining methods. A neuronal tract is a bundle of axons that connects neuropil regions. Tracts formed by a large number of axons, in particular large diameter ones, are easily delineated, but this may not be so when the number of axons is small.
Dense neuropils are further grouped into small modular structures of different shapes such as spherical, columnnar, layered. The word, module reminds a column in cerebral cortex of vertebrate. Although the vertebrate column can be distinguished functionally they are not compartmented morphologically (not true because there are the LAYERS! And for the horizontal coordinates, there is barrel cortex and the like, and binocular dominance columns can also be morphologically demonstrated!). On the other hand the insect module is functionally and morphologically compartmented so that it can be used in the study easily.
Modules in the insect nervous system are local microcircuits with similar properties supposedly arranged to process information in a parallel and distributed manner. For example a single lamina cartridge in the peripheral optic lobe of a fly processes information received by 6 photoreceptors looking at the same point in space whereas a glomerulus in the antennal lobe generally processes information from a population of olfactory receptor cells that express the same odorant receptor protein. Neuropil regions specifically concerned with a sensory modality (i.e. lamina of the optic lobe or antennal lobe) are built from such modules that are concerned with more fine-grained aspects within this modality (spatial distribution of light for the lamina, specific odorants for the antennal lobe) In other word one densed neuropile takes charge of one modality and each modules in the neuropile takes charge of a certain quality of modality
Brain structure and function
Insect brains consist of 105 to 106 neurons and brain neurons outnumber those composing the ventral nerve cord . A house fly brain includes about 350,000 neurons, that of a honeybee (worker) about 850,000. On the other hand each thoracic ganglion in any insect consists of 3000 to 5000 neurons and each abdominal ganglion of only 400 to 850 neurons .
The insect brain can be divided into three large regions, protocerebrum deutocerebrum, and tritocerebrum. As mentioned above each of them is associated with one of the three anteriormost ancestral body segments . [the use of “frontal” is always a bit dubious, but especially in insects.
The protocerebrum bears to lateral protrusions, the optic lobes. . Depending on the insect species, the optic lobe can be further subdivided into three or four neuropils. Medially,inbetween the optic lobes are located another conspicuous pair of bilaterally symmetrical neuropils, the mushroom bodies. . The median protocerebrum also contains unpaired neuropils, in particular the protocerebral bridge and the central body, which together form the central complex. The shape, size, and disposition of these dense neuropils differs somewhat between insect groups but the basic morphology is essentially the same. The optic lobes process visual information, the mushroom bodies are important for learning and memory related in particular to olfactory stimuli, and the central complex is thought to play a crucial role in generating behavioral output. The dense, clearly delineated neuropils are surrounded by neuropil areas without conspicuous structural characteristics.
The deutocerebrum is a bilaterally symmetrical brain region that consists of the antennal lobe and the dorsal lobe. The antennal lobe receives the axons of olfactory receptor neurons from the antenna . In at least some insects such as flies and moths, the antennal lobe also receives olfactory receptor axons from the labium. The dorsal lobe receives mechanosensory and gustatory receptor neurons from the antenna and also contains the motor neurons controlling the antennal muscles. The dorsal lobe is called antennal mechanosensory and motor center (AMMC) in some insects. For flies, this name may be retained because they differ from almost all other insects in not having an antennal gustatory system. The anntennal nerve is carrying all sensory axons from the antenna to the deutocerebrum and also contains efferent neurons, in particular the motor neurons of the pedicellus muscles. Generally, a second nerve emanates from beneath the antennal lobe that contains the axons of the motor neurons of the scapus muscles, which are located in the head capsule.
Tritocerebrum is the smallest region and little studied. In the primitive insect ground plan, the tritocerebrum is connected to the suboesophageal ganglian by a pair of circumoesophageal connectives (for example in locusts, crickets, cockroaches), in more derived groups, the tritocerebrum, the posterior part of the deutocerebrum, and the suboesophageal ganglion are fused such that it can be difficult to determine the borders between them. The tritocerebrum contains at least two commissures, one of which is running above the oesophagus in the primitive ground plan. The tritocerebrum receives sensory inputs from the labrum through the labral nerve, from the tegument through the tegumentary nerve, and at least in some insects also direct sensory input from the mouthparts (mandible, maxilla, and labium). The frontal nerves of the tritocerebra on each side run to the frontal ganglion that connects the brain and the stomatogastic system, lying dorsal with respect to the oesophagus and innervating cardiac and gastrointestinal muscles. The frontal ganglion is connected to the hypocerebral ganglion thourgh the recurrent nerve.In some insects (honeybee), the frontal and labral nerves are fused at the root to form a labro-frontal nerve. Tritocerebrum is connected to suboesophageal ganglion through a pair of connective. Frontal ganglion is center of stomatogastric ganglion innervating cardiac and gastrointestinal muscle and is connected to suboesophageal ganglion via recurrent nerve.
The suboesophageal ganglion is largely concerned with the processing of sensory information from and the motor control of the mouth parts. The mouth parts consist of three appendages, the mandible, the maxilla, and the labium. These appendages can be modified in various ways in different insect groups. For instance, many insects have paired maxillary and labial palps. In the bee, a part of the labium forms the unpaired glossae Axons of the receptor cells of the sensilla distributed on the mouth-parts project to the suboesophageal ganglion and in at least some insects also to higher areas. The suboesophageal ganglion also receives direct sensory input from the labrum and through non-olfactory sensory cells of the antenna. Generally, there are separate mandibular, maxillary, and labial nerves innervating the appendages (for example in the bee), but they can also be fused (such as in Dipera, in which there is a fused maxillary-labial nerve) At least in the bee, a little-known fourth nerve emanates from the labial neuromere: the labial gland nerve.
The thoracic ganglia possess motor centers that control important behaviors, such as flight, walking, and vocalization.
The abdominal ganglia possess motor centers related to posture control, rhythmic behavior, such as respiration, and circulation and the control of copulation and egg laying.
The motor centers residing in thoracic or abdominal ganglia autonomously express and correct motor patterns in response to their own local sensory inputs (trigger inputs) largely without depending on inputs from the brain. However, the initiation and maintenance of their motor patterns are generally controlled by descending interneurons from the brain.
The higher order neuropils in protocerebrum, such as mushroom body, central complex, and lateral protocerebrum, receive sensory information from optic lobe, deutocerebrum, tritocerebrum, suboesophageal ganglion, and ventral nerve cord through several layers of filters in a preprocessed form. The higher neuropils integrate information from various modalities and relay outputs that can eventually results in activating output neurons such as motor neurons of the brain or descending interneurons transmitting command information to the ventral nerve cord, which is important in controlling a wide range of behaviors. Thus, overall control mechanisms such as for triggering particular behaviors are localized in the brain and are separated from local control mechanisms that are responsible for aspects like the maintenance of motor patters for wing flapping or walking are localized in the thoracic ganglia. This is not to say that the ventral nerve cord is a fully hardwired system: In seminal experiments, Horridge could demonstate that cockroaches and locusts are capable of leg position learning, which is even improved by removal of the head. Thus, plasticity is possible at short time scales even in thoracic circuits controlling very basic behaviors.
Neuronal composition of the insect brain
Insect brain consists of neurons and glia cells. Both neuron and glia cell are differentiated from the same stem cells. Glia cells have traditionally been mostly seen as structural components, but there is no doubt that they also have important functional roles, which are still poorly understood in the insect brain.
Neurons (nerve cells) play the major role in information processing in the brain. Ethymologically, “neuron” is Greek for “string”, “tendon”, “nerve”, i. e. a term for elongated objects.
In the mammalian brain, glia cells are ten times more numerous than neurons whereas in the insect brain, the ratio is approximatedly reversed (Reference 1).
The basic structure and function of neurons is conserved across animal species. Simple nervous systems first emerged in Cnidaria, such as sea anemones and jellyfish, and evolved from loose nerve nets to compact centers, ganglia, which by further fusion and increase of complexity led to strctures one calls brains. From the evolutionary point of view insects represent a branch different from that to which humans belong. Nevertheless, their neurons have many functional properties in common. Therefore, the differences in the performance and capabilities between insect and human brain mainly arise from a difference in size and complexity. Insect brain contains 105 to 106 neurons whereas human brain harbours some 1011 neurons.
Insect neurons may be categorized into five types:
1) Sensory neuron: innervating sensory organs mostly in the cuticle, project from the periphery into the central nervous system.
2)Projection neuron: connects two or more separate regions in the central nervous system.
3)Local interneuron: confined to a certain region in the central nervous system.
4)Motor neuron: projects from central nervous system to muscle targets.
5)Neuroendocrine cell: innervates an endocrine organ.
Among these neuron types, only sensory neurons have somata localised outside the rind cortex (outer soma layer) of the central nervous system. This placement of somata is a prominent feature of the insect brain (and other arthopod brains). In the mammalian cortex for example, the gray matter contains somata, neurites, and synaptic contacts. In insects, the neuropil contains neurites and synapses and the somata are almost completely located in the rind, where synaptic contacts are exceptional.
Many mammalian neurites are myelinated, i.e. ensheathed by glia cells whereas many insect neurites are generally not myelinated. Insect glia cells often envelop bundles of neurites (such as tracts) or neuropil compartments, effectively forming borders.
Many neurons in insect brains are uniquely characterized by their morphology and function and are thus called identified neurons (Fig. 3). The fact that the insect brain possesses a comparatively small number of neurons most of which are identifiable allows us to study the insect brain at the cellular level. An extreme in terms of identified neurons is the nematode Caenorhabditis elegans, in which all 302 neurons have already been identified and even their lineage has been elucidated.
Dendrites are neurites that represent the predominant input regions of a neuron, receiving information from other neurons, mostly through synaptic contacts. In mammals, dendrites directly originate from the soma whereas in insect central neurons, only a primary neurite is sent off into the neuropil by the soma. The site of synapse can be inferred from the morphology of dendrite of a neuron. In many case dendritic spine (Insect neuron possesses spine?) is an input site whereas dendritic bouton and varicose are output sites. In addition dendrite near soma often resides input synapses whereas dendrite far from soma does output synapses. I find it a little confusing.
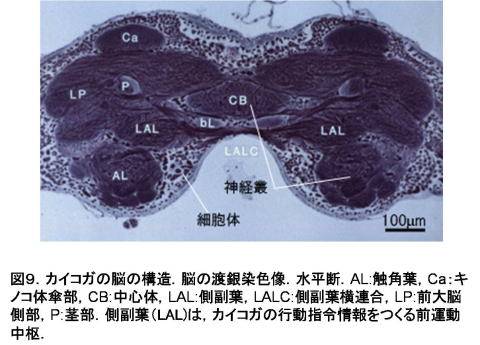
Figure 9. The structure of silkmoth brain (reduced silver stained horizontal section). AL, antennal lobe; Ca. calyx of the mushroom body; CB, central body; LAL, lateral accessory lobe; LALC, lateral accessory lobe commissure, LP, lateral protocerebrum; P, peduncle of the mushroom body. The LAL is an important premotor center generating command information.
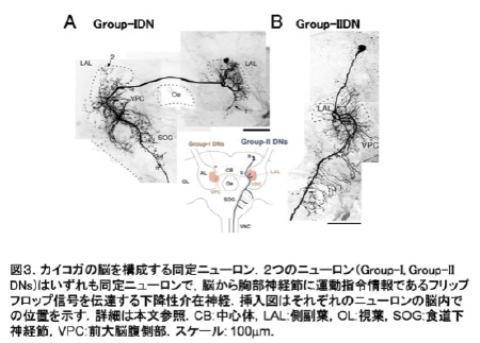
Figure 3. Identified neurons from the silkmoth brain. (A) Group-1 descending neuron (DN). (B)Group-2 DN. Group-1, and -2 DNs are both identified neurons that transmit a premotor command, the flip-flop signal, to the thoracic ganglia. The schematic illustration indicates the location of the somata of Group-1 and-2 DNs.
Morphology of neurons in the insect brain
Figure 8 shows examples of neurons in the insect brain. The spherical structure containing the nucleus is called soma (cell body). The size of somata is about 10 μm in diameter in many neurons (but varies considerably depending on the cell type within a species and also accross species). From the soma protrusions extend: the neurites. Neurons with a single neurite are called monopolar cells (A). Those with two neurites are bipolar cells (B). Those with more than two neurites are multipolar cells. Most insect neurons are monopolar cells. Their primary neurite runs into the neuropil and forms complex branching patterns. In general, the longest neurite within a neuron is the axon and other neurites are dendrites. The axon is representing the output channel of a neuron. On the axon, the output is relayed to other neurons through synapses, the axon being the output element. On the other side of a synapse, a dendrite, the input region of another neuron, receives this output. The patterns of connectivity of synapses between neurons results in a complex network for information processing.

urther reading
Insect Mimetics (2009) Shimozawa and Hariyama eds. NTS Japan
With contributions from S.S. Haupt and A. Takashima